Photonic Crystal Fibers
Acronym: PCF (also used for polymer cladded fibers!)
Definition: specialty optical fibers with a built-in microstructure, in most cases consisting of small air holes in glass
Alternative terms: microstructure fibers, holey fibers
More general term: optical fibers
More specific terms: photonic bandgap fibers, hollow-core fibers
German: mikrostrukturierte Fasern
Category: fiber optics and waveguides
How to cite the article; suggest additional literature
Author: Dr. Rüdiger Paschotta
Pioneered by the research group of Philip St. J. Russell in the 1990s, the development of photonic crystal fibers and the exploration of the great variety of possible applications have attracted huge interest. The field, which constitutes a part of the wider field of photonic bandgap structures while incorporating other ideas as well, can be considered as one of the most active fields of current optics research. This is partly because these fibers offer many degrees of freedom in their design to achieve a variety of peculiar properties, which make them interesting for a wide range of applications (see below).
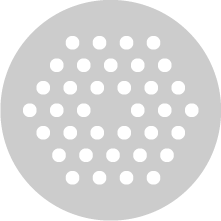
A photonic crystal fiber (also called holey fiber, hole-assisted fiber, microstructure fiber, or microstructured fiber) is an optical fiber which obtains its waveguide properties not from a spatially varying glass composition but from an arrangement of very tiny and closely spaced air holes which go through the whole length of fiber. Such air holes can be obtained by using a preform with (larger) holes, made e.g. by stacking capillary and/or solid tubes (stacked tube technique) and inserting them into a larger tube. Usually, this preform is then first drawn to a cane with a diameter of e.g. 1 mm, and thereafter into a fiber with the final diameter of e.g. 125 μm. Particularly soft glasses and polymers (plastics) also allow the fabrication of preforms for photonic crystal fibers by extrusion [13, 33]. There is a great variety of hole arrangements, leading to PCFs with very different properties. All these PCFs can be considered as specialty fibers.
The simplest (and most often used) type of photonic crystal fiber has a triangular pattern of air holes, with one hole missing (see Figure 1), i.e. with a solid core surrounded by an array of air holes. The guiding properties of this type of PCF can be roughly understood with an effective index model: the region with the missing hole has a higher effective refractive index, similar to the core in a conventional fiber.
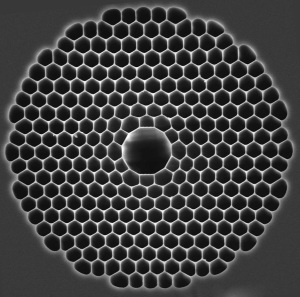
There are also so-called photonic bandgap fibers (PBG fibers) [6] with a totally different guiding mechanism, based on a photonic bandgap of the cladding region. The latter mechanism even allows guidance in a hollow core (i.e. in a low-index region) (see Figure 2), such that most of the power propagates in the central hole (→ hollow-core fibers). Such air-guiding hollow-core photonic crystal fibers (or air core bandgap fibers) can have a very low nonlinearity and a high damage threshold. They typically guide light only in a relatively narrow wavelength region with a width of e.g. 100–200 nm and can be used e.g. for pulse compression with high optical intensities, as most of the power propagates in the hollow core.
Most PCFs are made of pure fused silica (→ silica fibers), which is compatible with the above-mentioned fabrication techniques. However, various PCFs made of other materials have been demonstrated, most notably of heavy metal soft glasses and of polymers (plastic optical fibers), sometimes used even for terahertz radiation [12].
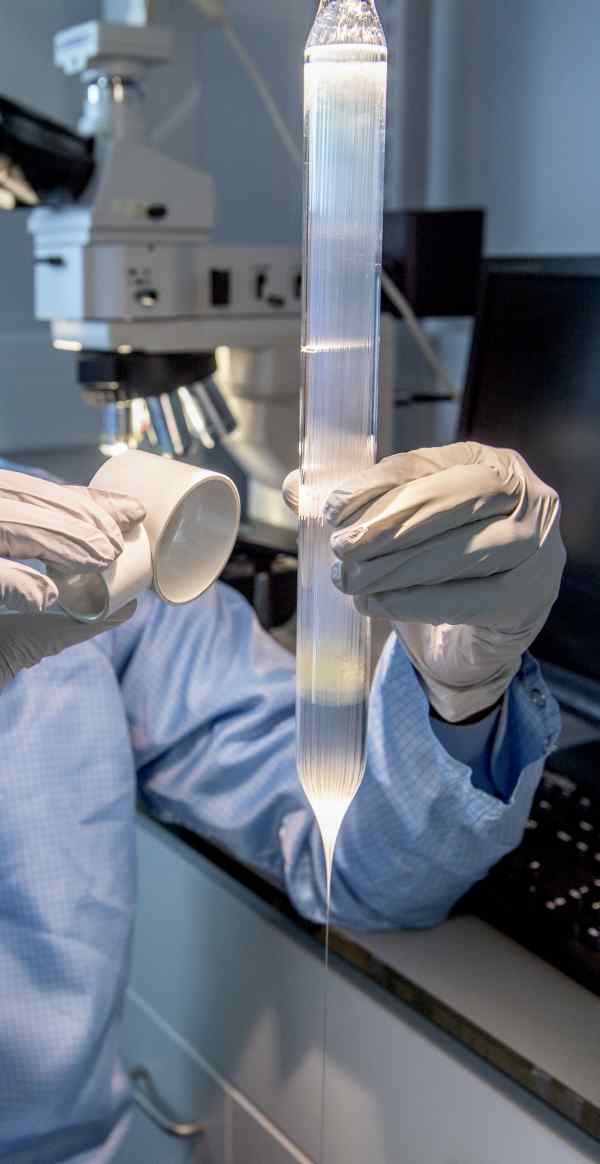
Active Fibers for Amplifiers and Lasers
Laser-active PCFs for fiber lasers and amplifiers can be fabricated, e.g., by using a rare-earth-doped rod as the central element of the preform assembly. Rare earth dopants (e.g. ytterbium or erbium) tend to increase the refractive index, but this can be precisely compensated, e.g. with additional fluorine doping, so that the guiding properties are determined by the photonic microstructure only and not by a conventional-type refractive index difference. With rare-earth-doped PCFs, it is possible to realize, e.g., soliton mode-locked fiber lasers operating in the 1-μm region, where a fiber's chromatic dispersion would usually be in the normal dispersion regime, but can be anomalous for suitable designs [7, 15, 16].
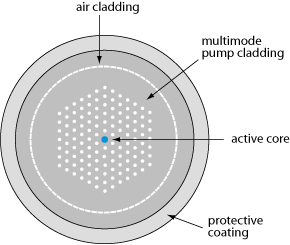
For high-power fiber lasers and amplifiers, double-clad PCFs (Figure 4) can be used, where the pump cladding is surrounded by an air cladding region (air-clad fiber). Due to the very large contrast of refractive index, the pump cladding can have a very high numerical aperture (NA), which significantly lowers the requirements on the pump source with respect to beam quality and brightness. Such PCF designs can also have very large mode areas of the fiber core [4, 26] while guiding only a single mode for diffraction-limited output, and are thus suitable for very high output powers with excellent beam quality. Another advantage is that the pump light is kept away from any polymer coating, thus avoiding possible problems with overheating of a coating.
Doped photonic crystal fibers have favorable properties also for use in fiber-based chirped-pulse amplification systems with very high output peak power.
Mode Profiles
In most cases, photonic crystal fibers either have single-mode propagation only or support a small number of guided modes (few-mode fibers). The intensity profiles of the fundamental guided modes are largely concentrated in the core, while higher-order modes tend to extend more into the cladding area. Normally, the mode fields extend into the air holes only to a very small amount; the intensity pattern are “squeezed” between the holes.
The calculation of mode profiles of such fibers is relatively difficult, (a) because no radial symmetry is given, as for most all-glass fibers, and (b) because of the large refractive index contrast between air and glass. Advanced mode solver software is required, which generally requires substantially longer computation times than simple mode solvers which are restricted to LP modes.
Properties Achievable by Design
Photonic crystal fibers with different designs of the hole pattern (concerning the basic geometry of the lattice, the relative size of the holes, and possibly small displacements) can have very remarkable properties, strongly depending on the design details:
- It is possible to obtain a very high numerical aperture of e.g. 0.6 or 0.7 of multimode fibers (also for the pump cladding of a double-clad fiber) [20].
- Single-mode guidance over very wide wavelength regions (endlessly single-mode fiber) is obtained for small ratios of hole size and hole spacing [3].
- Extremely small or extremely large mode areas (possibly with a lower NA than possible with a conventional fiber) are possible. These lead to very strong or very weak optical nonlinearities. PCFs can be made with a low sensitivity to bend losses even for large mode areas [4].
- Certain hole arrangements result in a photonic bandgap (→ photonic bandgap fibers), where guidance is possible even in a hollow core, as a higher refractive index in the inner part is no longer required. Such air-guiding hollow-core fibers are interesting e.g. for dispersive pulse compression at high pulse energy levels.
- Particularly for larger holes, there is the possibility to fill gases or liquids into the holes. Gas-filled PCFs can be exploited for fiber-optic sensors, for nonlinear spectral broadening at very high power levels, or for variable power attenuators.
- Asymmetric hole patterns can lead to extremely strong birefringence for polarization-maintaining fibers [8]. This can also be combined with large mode areas.
- Strongly polarization-dependent attenuation (polarizing fibers) [18, 24] can be obtained in different ways. For example, there can be a polarization-dependent fundamental mode cut-off, so that the fiber guides only light with one polarization in a certain wavelength range.
- Similarly, it is possible to suppress Raman scattering [25] by strongly attenuating longer-wavelength light.
- Very unusual chromatic dispersion properties, e.g. anomalous dispersion in the visible wavelength region [7], result particularly for PCFs with small mode areas. There is substantial design freedom, allowing for different combinations of desirable parameters.
- Core-less end caps can be fabricated simply by fusing the holes near the fiber end with a heat treatment. The sealed end facets allow for larger mode areas at the fiber surface and thus a higher damage threshold, e.g. for amplifying intense nanosecond pulses.
- Multicore designs are possible, e.g. with a regular pattern of core structures in a single fiber, where there may or may not be some coupling between the cores.
Technical Issues with Fiber Ends
Overall, photonic crystal fibers are handled in similar ways as standard optical fibers. However, special care is required in various respects:
- Ends of PCFs may not be cleaned with liquid solvents, such as ethanol, as capillary forces may pull them into the hole. Of course, the guiding properties can be strongly modified with any liquid in the holes. There is even research on exploiting such effects, e.g. for generating a tunable amount of optical loss by controlling the degree to which a liquid penetrates the holes.
- Cleaving and fusion splicing PCFs is possible, but can be more difficult, particularly for fibers with large air content. During fusion splicing, the air may expand and distort the fiber structure. Connections between fibers are also possible with a variety of mechanical splices, fiber connectors, protected patch cables, beam expansion units, etc.
- Even when the splicing process works well, there may be a substantial coupling loss due to a mismatch of mode areas, e.g. when a small-core PCF is coupled to a standard single-mode fiber. There are special tapered single-mode fibers and tapered PCFs for enhancing the coupling efficiency, but these may not be easily available.
Applications
Their special properties make photonic crystal fibers very attractive for a very wide range of applications. Some examples are:
- fiber lasers and amplifiers, including high-power devices, mode-locked fiber lasers, etc.
- nonlinear devices e.g. for supercontinuum generation [9, 28] (→ frequency combs), Raman conversion [11], parametric amplification, or pulse compression
- telecom components, e.g. for dispersion control, filtering or switching
- fiber-optic sensors of various kinds
- quantum optics, e.g. generation of correlated photon pairs [23], electromagnetically induced transparency, or guidance of cold atoms.
Even though PCFs have been around for several years, the huge range of possible applications is far from being fully explored. It is to be expected that this field will stay very lively for many years and many opportunities for further creative work, concerning both fiber designs and applications.
Suppliers
The RP Photonics Buyer's Guide contains 12 suppliers for photonic crystal fibers. Among them:
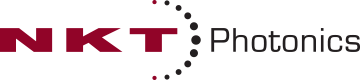
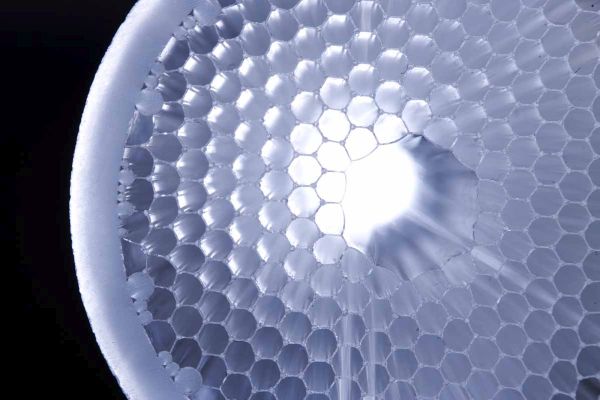
NKT Photonics
Our Crystal Fibre portfolio of specialty fibers spans from nonlinear fibers optimized for octave spanning supercontinuum generation, over the World’s largest single mode ytterbium gain fibers for high power lasers and amplifiers, to advanced hollow core fibers guiding the light in air. Our single-mode LMA fibers are also available as patch cords with standard termination in our aeroGUIDE product range.
Questions and Comments from Users
Here you can submit questions and comments. As far as they get accepted by the author, they will appear above this paragraph together with the author’s answer. The author will decide on acceptance based on certain criteria. Essentially, the issue must be of sufficiently broad interest.
Please do not enter personal data here; we would otherwise delete it soon. (See also our privacy declaration.) If you wish to receive personal feedback or consultancy from the author, please contact him e.g. via e-mail.
By submitting the information, you give your consent to the potential publication of your inputs on our website according to our rules. (If you later retract your consent, we will delete those inputs.) As your inputs are first reviewed by the author, they may be published with some delay.
Bibliography
[1] | P. Kaiser and H. W. Astle, “Low-loss single-material fibers made from pure fused silica”, Bell Syst. Tech. J. 53, 1021 (1974), doi:10.1002/j.1538-7305.1974.tb02780.x |
[2] | J. C. Knight et al., “All-silica single-mode optical fiber with photonic crystal cladding”, Opt. Lett. 21 (19), 1547 (1996), doi:10.1364/OL.21.001547 |
[3] | T. A. Birks et al., “Endlessly single-mode photonic crystal fibre”, Opt. Lett. 22 (13), 961 (1997), doi:10.1364/OL.22.000961 |
[4] | J. C. Knight et al., “Large mode area photonic crystal fibre”, Electron. Lett. 34, 1347 (1998), doi:10.1049/el:19980965 |
[5] | D. Mogilevtsev et al., “Group-velocity dispersion in photonic crystal fibres”, Opt. Lett. 23 (21), 1662 (1998), doi:10.1364/OL.23.001662 |
[6] | R. F. Cregan et al., “Single-mode photonic band gap guidance of light in air”, Science 285, 1537 (1999), doi:10.1126/science.285.5433.1537 |
[7] | J. C. Knight et al., “Anomalous dispersion in photonic crystal fiber”, IEEE Photon. Technol. Lett. 12, 807 (2000), doi:10.1109/68.853507 |
[8] | A. Ortigosa-Blanch et al., “Highly birefringent photonic crystal fibres”, Opt. Lett. 25 (18), 1325 (2000), doi:10.1364/OL.25.001325 |
[9] | J. K. Ranka et al., “Visible continuum generation in air–silica microstructure optical fibers with anomalous dispersion at 800 nm”, Opt. Lett. 25 (1), 25 (2000), doi:10.1364/OL.25.000025 |
[10] | T. A. Birks et al., “Supercontinuum generation in tapered fibers”, Opt. Lett. 25 (19), 1415 (2000), doi:10.1364/OL.25.001415 |
[11] | F. Benabid et al., “Stimulated Raman scattering in hydrogen-filled hollow-core photonic crystal fiber”, Science 298, 399 (2002), doi:10.1126/science.1076408 |
[12] | H. Han et al., “Terahertz pulse propagation in a plastic photonic crystal fiber”, Appl. Phys. Lett. 80 (15), 2634 (2002), doi:10.1063/1.1468897 |
[13] | V. V. Ravi Kanth Kumar et al., “Extruded soft glass photonic crystal fiber for ultrabroad supercontinuum generation”, Opt. Express 10 (25), 1520 (2002), doi:10.1364/OE.10.001520 |
[14] | W. H. Reeves et al., “Transformation and control of ultrashort pulses in dispersion-engineered photonic crystal fibres”, Nature 424, 511 (2003), doi:10.1038/nature01798 |
[15] | J. C. Knight, “Photonic crystal fibres”, Nature 424, 847 (2003), doi:10.1038/nature01940 |
[16] | P. St. J. Russell, “Photonic crystal fibers” (review paper), Science 299, 358 (2003), doi:10.1126/science.1079280 |
[17] | D. G. Ouzounov et al., “Generation of megawatt optical solitons in hollow-core photonic band-gap fibers”, Science 301, 1702 (2003), doi:10.1126/science.1088387 |
[18] | D. A. Nolan et al., “Single-polarization fiber with a high extinction ratio”, Opt. Lett. 29 (16), 1855 (2004), doi:10.1364/OL.29.001855 |
[19] | K. Hougaard and F. D. Nielsen, “Amplifiers and lasers in PCF configurations”, J. Opt. Fiber Commun. Rep. 1, 63–83 (2004), doi:10.1007/s10297-004-0018-9 |
[20] | W. J. Wadsworth et al., “Very high numerical aperture fibers”, IEEE Photon. Technol. Lett. 16, 843 (2004), doi:10.1109/LPT.2004.823689 |
[21] | P. J. Roberts et al., “Ultimate low loss of hollow-core photonic crystal fibres”, Opt. Express 13 (1), 236 (2005), doi:10.1364/OPEX.13.000236 |
[22] | K. Saitoh and M. Koshiba, “Empirical relations for simple design of photonic crystal fibers”, Opt. Express 13 (1), 267 (2005), doi:10.1364/OPEX.13.000267 |
[23] | J. G. Rarity et al., “Photonic crystal fiber source of correlated photon pairs”, Opt. Express 13 (2), 534 (2005), doi:10.1364/OPEX.13.000534 |
[24] | T. Schreiber et al., “Stress-induced single-polarization single-transverse mode photonic crystal fiber with low nonlinearity”, Opt. Express 13 (19), 7621 (2005), doi:10.1364/OPEX.13.007621 |
[25] | L. A. Zenteno et al., “Suppression of Raman gain in single-transverse-mode dual-hole-assisted fiber”, Opt. Express 13 (22), 8921 (2005), doi:10.1364/OPEX.13.008921 |
[26] | W. S. Wong et al., “Breaking the limit of maximum effective area for robust single-mode propagation in optical fibers”, Opt. Lett. 30 (21), 2855 (2005), doi:10.1364/OL.30.002855 |
[27] | J. M. Fini, “Aircore microstructure fibers with suppressed higher-order modes”, Opt. Express 14 (23), 11354 (2006), doi:10.1364/OE.14.011354 |
[28] | J. M. Dudley et al., “Supercontinuum generation in photonic crystal fiber”, Rev. Mod. Phys. 78, 1135 (2006), doi:10.1103/RevModPhys.78.1135 |
[29] | P. St. J. Russell, “Photonic-crystal fibers”, J. Lightwave Technol. 24 (12), 4729 (2006), doi:10.1109/JLT.2006.885258 |
[30] | G. Ren et al., “Low-loss all-solid photonic bandgap fiber”, Opt. Lett. 32 (9), 1023 (2007), doi:10.1364/OL.32.001023 |
[31] | Y. Tsuchida et al., “Design of single-moded holey fibers with large-mode-area and low bending losses: the significance of the ring-core region”, Opt. Express 15 (4), 1794 (2007), doi:10.1364/OE.15.001794 |
[32] | H. Ebendorff-Heidepriem et al., “Fluoride glass microstructured optical fiber with large mode area and mid-infrared transmission”, Opt. Lett. 33 (23), 2861 (2008), doi:10.1364/OL.33.002861 |
[33] | H. Ebendorff-Heidepriem et al., “Suspended nanowires: fabrication, design and characterization of fibers with nanoscale cores”, Opt. Express 17 (4), 2646 (2009), doi:10.1364/OE.17.002646 |
[34] | Z. Chen et al., “More than threefold expansion of highly nonlinear photonic crystal fiber cores for low-loss fusion splicing”, Opt. Lett. 34 (14), 2240 (2009), doi:10.1364/OL.34.002240 |
[35] | C. Markos et al., “Hybrid photonic-crystal fiber”, Rev. Mod. Phys. 89, 045003 (2017), doi:10.1103/RevModPhys.89.045003 |
See also: fibers, rare-earth-doped fibers, photonic bandgap fibers, hollow-core fibers, tapered fibers, supercontinuum generation, frequency combs
and other articles in the category fiber optics and waveguides

This encyclopedia is authored by Dr. Rüdiger Paschotta, the founder and executive of RP Photonics Consulting GmbH. How about a tailored training course from this distinguished expert at your location? Contact RP Photonics to find out how his technical consulting services (e.g. product designs, problem solving, independent evaluations, training) and software could become very valuable for your business!
![]() |
If you like this page, please share the link with your friends and colleagues, e.g. via social media:
These sharing buttons are implemented in a privacy-friendly way!