Narrow-linewidth Lasers
Definition: single-frequency lasers with a narrow optical emission spectrum
More general terms: lasers
German: Laser mit geringer Linienbreite, schmalbandige Laser
Categories: lasers, fluctuations and noise
How to cite the article; suggest additional literature
Author: Dr. Rüdiger Paschotta
A number of laser applications (see below) require lasers with a very small optical linewidth, i.e., with a narrow optical spectrum. The term narrow-linewidth lasers usually applies to single-frequency lasers, i.e., lasers oscillating on a single resonator mode with low phase noise and thus with high spectral purity. Typically, such lasers also exhibit low intensity noise.
Types of Narrow-linewidth Lasers
The most important types of narrow-linewidth lasers are the following:
- Among semiconductor lasers, distributed feedback laser diodes (DFB lasers) and distributed Bragg reflector lasers (DBR lasers), operating e.g. in the 1.5- or 1.0-μm wavelength region, are the most common. Typical operation characteristics are an output power of some tens of milliwatts (or possibly somewhat above 100 mW) and a linewidth of several megahertz.
- Significantly smaller linewidths from semiconductor lasers are possible e.g. by extending the resonator with a single-mode fiber containing a narrow-bandwidth fiber Bragg grating, or with other types of external-cavity diode lasers. In such a way, ultra-narrow linewidths of a few kilohertz or even below 1 kHz can be achieved.
- Small fiber lasers in the form of distributed feedback lasers (with the resonator formed essentially by a special fiber Bragg grating) can generate tens of milliwatts of output power with a linewidth in the region of a few kilohertz.
- Higher output powers can be generated with longer distributed Bragg reflector lasers (DBR fiber lasers) or unidirectional fiber ring lasers, and also by using a fiber amplifier.
- Diode-pumped solid-state bulk lasers, e.g. in the form of nonplanar ring oscillators, can also have linewidths of a few kilohertz, combined with relatively high output powers of the order of 1 W. Although a 1064-nm wavelength is typical, other wavelengths e.g. in the 1.3- or 1.5-μm wavelength regions are also possible.
Essential Factors for a Narrow Laser Linewidth
For achieving a narrow emission bandwidth (linewidth) from a laser, several issues of laser design have to be observed:
- First, single-frequency operation needs to be achieved. This is easiest when using a gain medium with small gain bandwidth and a laser resonator with short length (leading to a large free spectral range). The goal should be long-term stable single-frequency operation without mode hopping.
- Second, external noise influences must be minimized. This requires a stable resonator setup (preferably a monolithic one), possibly with special protection against mechanical vibrations. An electrically pumped laser should be operated with a low-noise voltage or current source, and an optically pumped laser should have a pump source with low intensity noise. Furthermore, any optical feedback must be avoided, e.g. by using a Faraday isolator. Ideally, external noise influences will become lower than internal noise, e.g. from spontaneous emission in the gain medium. This is often easily achievable at high noise frequencies, but not at low noise frequencies which are most important for the linewidth.
- Third, the laser design should be optimized so that the laser noise and in particular the phase noise are minimized. A high intracavity optical power and long resonator can be beneficial, although stable single-frequency operation is more difficult to achieve with a longer resonator.
Of course, the design optimization requires that the relative importance of different noise sources is known, because different measures can be required depending on which noise source is dominant. For example, measures which minimize the linewidth according to the Schawlow–Townes equation will not necessarily minimize the actual linewidth, if this is determined e.g. by mechanical noise.
Noise Characterization and Specification
Both the characterization and the specification of the noise of narrow-linewidth lasers are far from trivial issues. Various measurement techniques are discussed in the article on linewidth, and particularly for linewidth values of a few kilohertz or less such measurements are demanding. Furthermore, a linewidth value alone can not be considered a complete noise characterization; it is preferable to have a complete phase noise spectrum, apart from information on relative intensity noise. At least, the linewidth value should be specified together with a measurement time, and possibly with some information concerning frequency drifts for longer time intervals.
Of course, different applications have different requirements, and it should be checked in detail how tight noise specifications should really be demanded in any particular case.
Applications of Narrow-linewidth Lasers
- A particularly important field of application is the area of sensors, e.g. fiber-optic sensors for strain and/or temperature, various types of interferometric sensing, trace gas detection with differential absorption LIDAR (DIAL), or wind speed measurements with Doppler LIDAR. Linewidths of only a few kilohertz are required for some fiber-optic sensors, whereas 100 kHz can be sufficient for, e.g., LIDAR measurements.
- Optical frequency metrology requires sources with very narrow linewidth, often achieved with stabilization techniques.
- Holography requires either continuous-wave or pulsed single-frequency lasers for generating highly coherent light.
- Normally less demanding in terms of linewidth are applications in optical fiber communications, e.g. in transmitters or for test and measurement purposes.
Suppliers
The RP Photonics Buyer's Guide contains 74 suppliers for narrow-linewidth lasers. Among them:

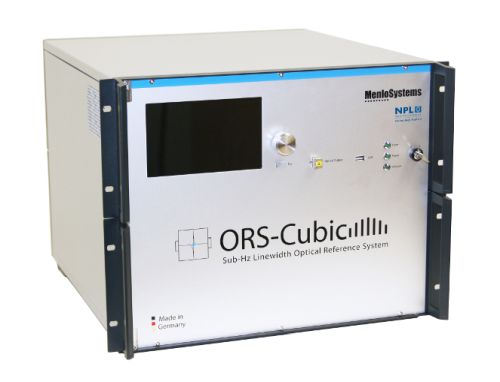
Menlo Systems
Menlo Systems offers ultrastable, frequency stabilized lasers at basically any wavelength. We supply fully characterized systems with linewidths <1 Hz and Allan deviations of 2 × 10-15 (in 1 s) as well as modules and components allowing for state-of-the-art systems tailored to your requirements.

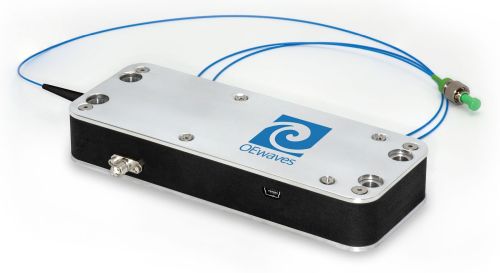
OEwaves
HI-Q™ 1.5 MICRON LASER: ultra-narrow Lorentzian linewidth less than 1 Hz and low phase/frequency noise in a compact form factor, emitting at 1530–1565 nm.
HI-Q™ 1 MICRON LASER: ultra-narrow Lorentzian linewidth less than 80 Hz and low phase/frequency noise in a compact form factor; 980–1080 nm.
HI-Q™ 2 MICRON LASER: ultra-narrow Lorentzian linewidth less than 80 Hz and low phase/frequency noise in a compact form factor; 1900–2100 nm.
HI-Q™ CUSTOM LASER: custom built laser with ultra-narrow Lorentzian linewidth and low phase/frequency noise in a compact form factor in the range 370–4500 nm.
Features:
- ultra-narrow instantaneous laser linewidth
- ultra-low ühase/frequency noise
- 370 – 4500 nm wavelength
- low vibration sensitivity
- low residual amplitude modulation
- wavelength stability
- compact package
- integrated driver/controller
- USB or RS232 control interface
Applications:
- interferometric optical sensing
- LIDAR
- B-OTDR temperature and strain
- gas sensing
- optical metrology and spectroscopy
- acoustic sensing
- oil and gas exploration
- coherent communication
- test and measurement
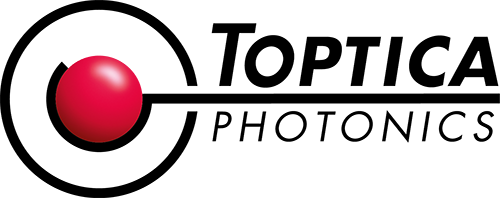
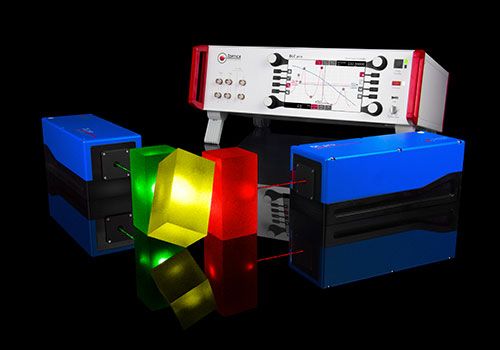
TOPTICA Photonics
All of TOPTICA’s tunable diode lasers offer a narrow linewidth of typically 100 kHz, corresponding to coherence lengths of almost 1 km. By stabilizing these lasers even further with TOPTICA’s locking electronics, linewidths below 1 Hz are possible.
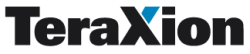
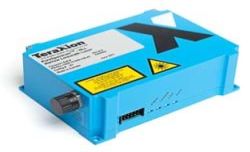
TeraXion
The PureSpectrum™ NLL is an ultra-low-noise, narrow linewidth semiconductor DFB laser for optical sensing applications like lidar and RF over fiber. The laser and its control electronics are integrated into a single module that uses precision feedback monitoring to provide ultra low-noise performance and superior wavelength stability. The built-in, sharp-edged frequency discriminator significantly reduces linewidth while maintaining single-mode operation across the full operating temperature range. The model also offers fast frequency tuning, which enables frequency-modulated continuous-wave sensing.

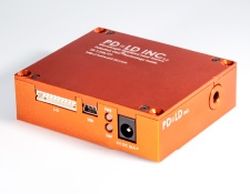
Edmund Optics
Edmund Optics offers narrow-linewidth lasers, e.g. in the form of metrology lasers with ±0.002 nm wavelength stability.
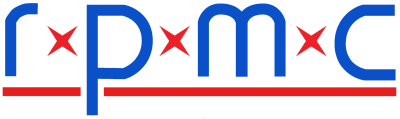
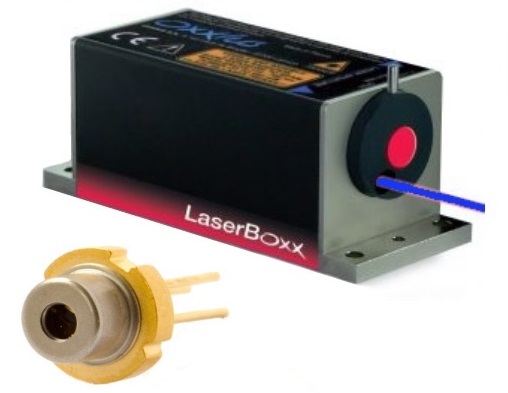
RPMC Lasers
RPMC offers a wide range of narrow linewidth laser diodes and laser modules with wavelengths from 405 nm to 2 μm. These offerings include DFB and DBR diode lasers, external cavity VBG diode lasers, fiber lasers, and DPSS lasers. All are available in both OEM and turnkey packages.
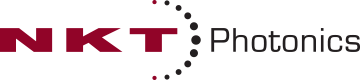
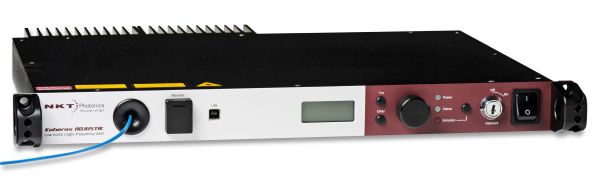
NKT Photonics
The Koheras narrow linewidth, single-frequency fiber lasers are ultra-low noise sources with longitudinal single mode and single frequency operation. The lasers are based on a DFB design ensuring robust and reliable operation and are delivered as fully integrated systems for industrial and scientific applications. Koheras offers an unprecedented low phase- and intensity noise level at Yb, Er and Tm wavelengths. It has a very high stability, and mode-hop free inherent single frequency output – even when exposed to changing environmental conditions. You can also get shot noise limited solutions for applications demanding an extra low intensity noise level.
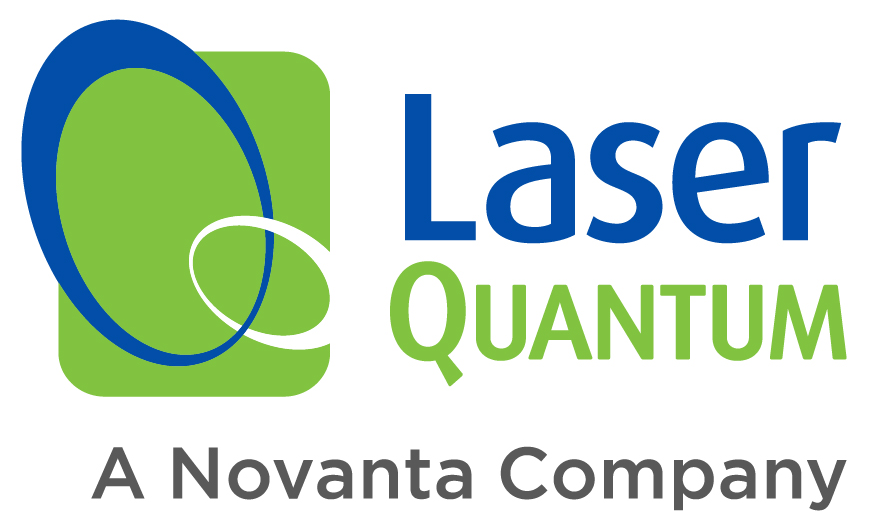
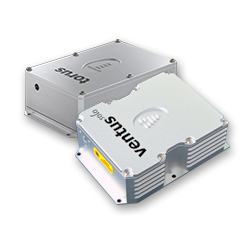
Laser Quantum
Laser Quantum offer a number of lasers with a narrow linewidth and high spectral purity, including the torus 532 and torus 660 mode-locked SLM lasers and the ventus solo, designed for high resolution Raman spectroscopy applications.
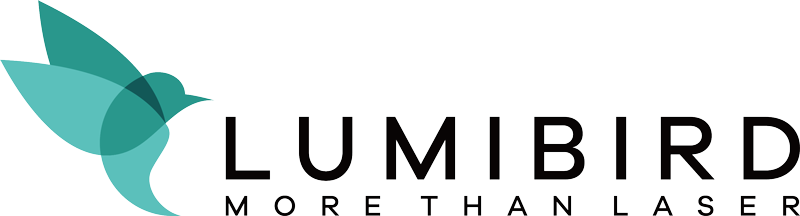
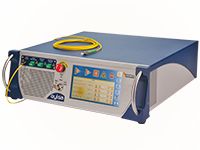
Lumibird
With the CVFL, CYFL and CEFL kilo models, Lumibird offers CW fiber lasers with very narrow linewidth down to 1 kHz. These single frequency lasers emits at 1054/1083 nm for the ytterbium version, in the 1.5-µm range for the erbium version and at frequency converted wavelengths for the CVFL model. These lasers are specifically designed for applications which requirehigh precision such as LIDAR, atomic spectroscopy, or atom cooling.
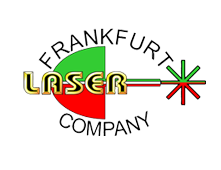
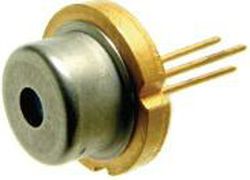
Frankfurt Laser Company
Frankfurt Laser Company offers various kinds of wavelength-stabilized laser diodes which all exhibit a narrow emission linewidth. Those based on DFB or DBR lasers even exhibit single-frequency operation.
Questions and Comments from Users
Here you can submit questions and comments. As far as they get accepted by the author, they will appear above this paragraph together with the author’s answer. The author will decide on acceptance based on certain criteria. Essentially, the issue must be of sufficiently broad interest.
Please do not enter personal data here; we would otherwise delete it soon. (See also our privacy declaration.) If you wish to receive personal feedback or consultancy from the author, please contact him e.g. via e-mail.
By submitting the information, you give your consent to the potential publication of your inputs on our website according to our rules. (If you later retract your consent, we will delete those inputs.) As your inputs are first reviewed by the author, they may be published with some delay.
Bibliography
[1] | M. Fleming and A. Mooradian, “Spectral characteristics of external-cavity controlled semiconductor lasers”, IEEE J. Quantum Electron. 17 (1), 44 (1981), doi:10.1109/JQE.1981.1070634 |
[2] | K. Kobayashi and I. Mito, “Single frequency and tunable laser diodes”, J. Lightwave Technol. 6 (11), 1623 (1988) |
[3] | S. P. Smith et al., “Narrow-linewidth stimulated Brillouin fiber laser and applications”, Opt. Lett. 16 (6), 393 (1991), doi:10.1364/OL.16.000393 |
[4] | N. Uehara and K. I. Ueda, “193-mHz beat linewidth of frequency-stabilized laser-diode-pumped Nd:YAG ring lasers”, Opt. Lett. 18 (7), 505 (1993), doi:10.1364/OL.18.000505 |
[5] | Y. Shevy and H. Deng, “Frequency-stable and ultranarrow-linewidth semiconductor laser locked directly to an atom-cesium transition”, Opt. Lett. 23 (6), 472 (1998), doi:10.1364/OL.23.000472 |
[6] | B. C. Young et al., “Visible lasers with subhertz linewidths”, Phys. Rev. Lett. 82 (19), 3799 (1999), doi:10.1103/PhysRevLett.82.3799 |
[7] | R. M. Williams et al., “Kilohertz linewidth from frequency-stabilized mid-infrared quantum cascade lasers”, Opt. Lett. 24 (24), 1844 (1999), doi:10.1364/OL.24.001844 |
[8] | St. A. Webster et al., “Subhertz-linewidth Nd:YAG laser”, Opt. Lett. 29 (13), 1497 (2004), doi:10.1364/OL.29.001497 |
[9] | J. Geng et al., “Narrow linewidth fiber laser for 100-km optical frequency domain spectroscopy”, IEEE Photon. Technol. Lett. 17 (9), 1827 (2005), doi:10.1109/LPT.2005.853258 |
[10] | A. Polynkin et al., “Single-frequency fiber ring laser with 1 W output power at 1.5 μm”, Opt. Express 13 (8), 3179 (2005), doi:10.1364/OPEX.13.003179 |
[11] | H. Stoehr et al., “Diode laser with 1 Hz linewidth”, Opt. Lett. 31 (6), 736 (2006), doi:10.1364/OL.31.000736 |
[12] | J. Geng et al., “Dual-frequency Brillouin fiber laser for optical generation of tunable low-noise radio frequency/microwave frequency”, Opt. Lett. 33 (1), 16 (2008), doi:10.1364/OL.33.000016 |
[13] | Y. Zhao et al., “Sub-Hertz frequency stabilization of a commercial diode laser”, Opt. Commun. 283, 4696 (2010), doi:10.1016/j.optcom.2010.06.079 |
[14] | S. Vogt et al., “Demonstration of a transportable 1 Hz-linewidth laser”, Appl. Phys. B 104 (4), 741 (2011), doi:10.1007/s00340-011-4652-7 |
[15] | A. Buikema et al., “Narrow-linewidth fiber amplifier for gravitational-wave detectors”, Opt. Lett. 44 (15), 3833 (2019), doi:10.1364/OL.44.003833 |
See also: linewidth, single-frequency lasers, laser applications, laser noise, noise specifications, laser spectroscopy
and other articles in the categories lasers, fluctuations and noise
![]() |
If you like this page, please share the link with your friends and colleagues, e.g. via social media:
These sharing buttons are implemented in a privacy-friendly way!