Modeling in Photonics
What is a Model? | Examples | Transparent Laser | Get started |
Examples for Models in Laser Technology
In the following, you find a few examples for the application of computer models in laser technology. See also our tutorial on modeling.
Light Amplification in Laser Crystals
It is well known that light can be amplified by stimulated emission e.g. in laser crystals. In a laser crystal, additional effects such as gain saturation, gain guiding and diffraction also come into play. But how do all these effects combine in detail? A clear quantitative understanding of such processes is indispensable for the efficient development of laser devices.
A model for the amplification of a laser beam in a bulk laser may contain the following:
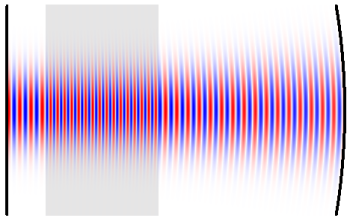
- The laser crystal is assumed to contain laser-active ions with a certain doping concentration.
- The internal working of the laser-active ions can be described with an energy level scheme and rate equations, containing parameters like wavelength-dependent transition cross sections and an upper-state lifetime.
- The laser beam is described by complex field amplitudes on a numerical grid which spans the laser crystal and possibly also other optical components (in some cases, a whole laser resonator).
A suitable software such as RP Fiber Power can numerically simulate the beam propagation through the laser crystal (or in many round trips through a laser resonator), taking into account stimulated emission, diffraction, gain guiding, saturable absorption, etc. (Of course, the user of such software does not need to deal with the details of how such things are calculated.) As an example, see a case study on an actively Q-switched laser.
Simpler models assume a fixed transverse profile of the laser beam and effectively propagate only optical powers rather than full beam profiles. They can be processed much faster, but only if one knows that beam profiles are not significantly affected e.g. by gain guiding effects.
Light Amplification in Active Fibers
Light can also be amplified in rare-earth-doped fibers. The essential physical foundations are the same as for laser crystals, but nevertheless some aspects can be quite different:
- In many cases, transverse beam profiles can be considered as totally determined by waveguide properties only. This greatly simplifies the propagation, because simple power propagation is sufficient (see our tutorial on modeling).
- In most cases, quasi-three-level laser transitions are used, where one needs to take into account reabsorption effects.
- The optical intensities are often very high, causing strong gain saturation effects as well as nonlinear effects.
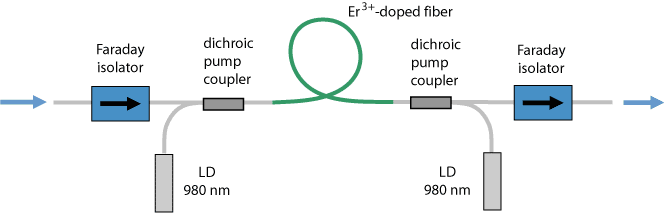
A suitable software such as RP Fiber Power can do efficient power propagation, or full beam propagation where needed. It can not only fully treat quasi-three-level systems, but even work with arbitrary user-defined energy level schemes.
Generation of Ultrashort Pulses in a Mode-locked Laser
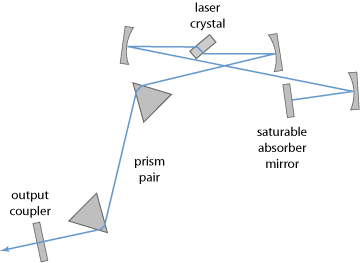
In a mode-locked laser, a single ultrashort pulse (or sometimes a sequence of pulses) circulates in the laser resonator. In each resonator round trip, it is subject to a variety of effects such as wavelength-dependent amplification and power losses, chromatic dispersion and nonlinearities, and to time-dependent losses in an optical modulator or a saturable absorber. Ideally, a steady state is reached after some number of round trips, so that the combined action of all mentioned effects exactly reproduces the pulse after each full round trip. In other cases, such a stable steady-state does not exist; instabilities can arise in very different ways, which are often hard to distinguish in experiments.
A model for a mode-locked laser may contain the following:
- The circulating pulse is represented by a numerical array a of complex amplitudes in the time or frequency domain. (Both can be related to each other by a Fast Fourier Transform.)
- Each optical component (for example, the laser crystal, dispersive laser mirrors, and modulator or a saturable absorber) is characterized by various quantities describing its effect on the circulating pulse.
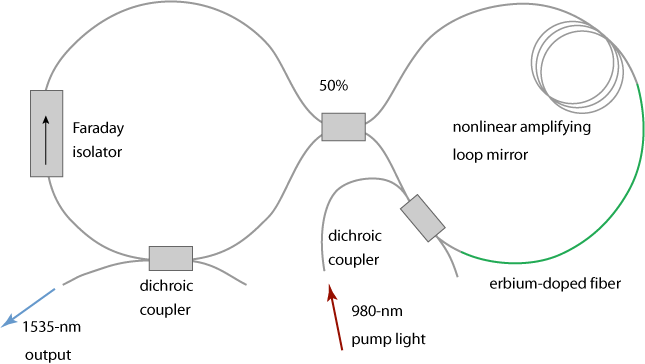
A suitable software such as RP ProPulse or RP Fiber Power can numerically simulate the evolution of the circulating pulse in many round trips. One can, for example, plot various pulse parameters as functions of the number of round trips, inspect the pulse traces in the time and frequency domain for any location and moment of time, or plot steady-state pulse parameters as functions of other parameters such as the pump power, the chromatic dispersion of an optical element or whatever else.
With such a tool, it is possible, for example, to
- calculate the steady-state pulse parameters and study their dependence on various device and operation parameters,
- analyze the importance of various potentially disturbing effects, and to
- investigate possible causes for instabilities.
Based on this, one can optimize the laser design for best performance and/or lowest sensitivity to various influences.
Particularly for fiber lasers, the interplay of various effects is usually so complex, that without a computer simulation (i.e., only based on a good understanding of the technology) one could not understand which pulse parameters are to be expected, what are the stability limits, etc.
Amplifier Systems for Ultrashort Pulses
For many applications, one requires ultrashort light pulses with high pulse energies and enormously high peak powers. Typically, one first generates low-energy pulses in a mode-locked laser, then uses a pulse picker which greatly lowers the pulse repetition rate, and finally sends that pulse train through a chain of optical amplifiers. Different types of amplifiers can be used, such as fiber amplifiers (providing a high gain, high amplification bandwidth and high power conversion efficiency, but exhibiting substantial nonlinear effects) and bulk amplifiers based on laser crystals.
Particularly in case of multi-stage amplifier systems, numerical simulations are indispensable for acquiring a reasonable understanding of what happens inside and how the system performance can be optimized. A lot of technical details can be relevant:
- In each amplifier stage, there may be substantial effects of gain saturation, gain narrowing (i.e., a reduction of optical bandwidth due to a limited gain bandwidth) and nonlinear distortions.
- High-gain systems also exhibit substantial amounts of amplified spontaneous emission (ASE). Often, one needs to prevent such ASE light as far as possible from entering the next or previous amplifier stage, e.g. in order to avoid excessive gain saturation by ASE. For such purposes, one may employ devices like bandpass filters, Faraday isolators and optical switches. Of course, their effects need to be properly treated in a model.
- In many cases, the principle of chirped-pulse amplification (CPA) is employed, using highly dispersive optical elements like volume Bragg gratings or pairs of diffraction gratings.
A suitable simulation software such as RP Fiber Power can not only calculate the characteristics of the output pulses even in complex situations (with multiple amplifier stages, ASE feedback effects, etc.), but also provides a high flexibility for varying any device parameters, studying dynamical effects, etc.
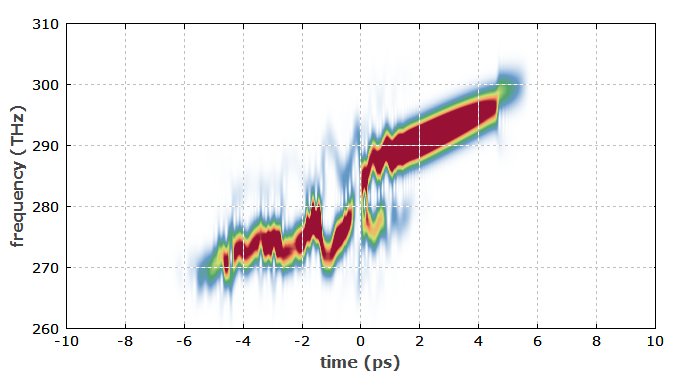
Further Examples
As further examples, you can take numerous case studies performed with some of our software products:
- RP Fiber Power (applicable to passive fibers, fiber lasers and amplifiers, often also to bulk lasers)
- RP Resonator (laser resonator design)
- RP ProPulse (pulse propagation, e.g. in mode-locked lasers and synchronously pumped optical parametric oscillators)
- RP Coating (design of thin-film devices)
Each one demonstrates that computer modeling reveals not only the expected performance, but also the internal details, which is essential for understanding and optimizing such devices.