Mode-locked Lasers
Definition: lasers which emit ultrashort pulses on the basis of the technique of mode locking
Alternative term: modelocked lasers
More general term: pulsed lasers
German: modengekoppelte Laser
Categories: lasers, light pulses
How to cite the article; suggest additional literature
Author: Dr. Rüdiger Paschotta
A mode-locked laser is a laser to which the technique of active or passive mode locking is applied, so that a periodic train of ultrashort pulses is emitted. See the articles on mode locking and mode locking devices for more details on mode-locking techniques; the present article focuses more on the lasers themselves. The article on ultrafast lasers also gives some idea about current developments in ultrashort pulse generation.
As ultrashort pulses have a certain bandwidth, mode-locked lasers for short pulses (particularly in the sub-picosecond region) require a gain medium with a large gain bandwidth. Other desirable features are a not too high nonlinearity and chromatic dispersion, and (particularly for passive mode locking) high enough laser cross sections in order to avoid Q-switching instabilities.
Types of Mode-locked Lasers
The following types of lasers are particularly attractive for mode locking:
- In the 1970s, dye lasers were routinely used, which were pumped with argon ion lasers. Laser dyes have a broad gain bandwidth, allowing for very short pulses. However, dye lasers have been largely replaced with solid-state lasers once these were able to deliver similar or better performance.
- Solid-state bulk lasers, based on ion-doped crystals or glasses, are today the dominant type of mode-locked lasers. They allow for very short pulses, very high pulse energies and/or average output powers, high or low pulse repetition rates, and high pulse quality. Some record achievements are listed below.
- Fiber lasers can also be mode-locked for generating very short pulses with potentially cheap setups. See the article on mode-locked fiber lasers for more details. High output powers are typically not achieved directly, but by using fiber amplifiers. The achieved pulse durations of ultrafast fiber lasers are often limited by nonlinearities or by higher-order dispersion, rather than by the gain bandwidth.
- Semiconductor lasers can be built as mode-locked diode lasers, mostly for applications in optical fiber communications. More recently, optically pumped passively mode-locked VECSELs have been demonstrated which can rival other solid-state lasers, particularly if a combination of relatively high output power, a multi-gigahertz pulse repetition rate, and possibly a short pulse duration (a few picoseconds or less) is required.
Due to the very different properties of those gain media, it is vital to select an appropriate medium for operation of a mode-locked laser in a particular parameter regime, e.g. concerning pulse duration, center wavelength and pulse repetition rate.
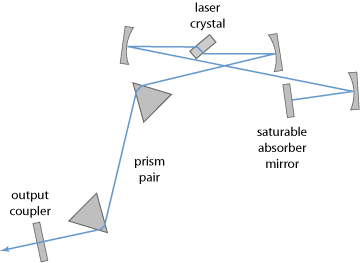
Design Issues
The design of a mode-locked laser is generally a non-trivial task, and particularly so if extreme parameter regions for the pulse parameters are targeted. There is a complicated interplay of many effects, including dispersion and several nonlinear effects, and changing one design parameter often influences several others. (For example, in a soliton mode-locked laser a change in mode size in the laser crystal or of the cavity length changes the balance of nonlinearity and dispersion, and thus also the pulse duration.) As a result, it can be difficult to achieve simultaneously very short pulses, stable operation, and a high power efficiency. For given parameters of the gain medium, there can be certain restrictions on the achievable pulse parameters. A relatively trivial one is that a gain medium with a small gain bandwidth is not suitable for generating very short pulses. Certainly more surprising is e.g. the finding that mode-locked solid-state lasers have difficulties in combining a high pulse repetition rate with a high average output power, and that the additional requirement of generating sub-picosecond pulses makes this trade-off even much more demanding. Such constraints arise from a combination of effects and issues such as Q-switched mode locking and other kinds of instabilities, details of pulse shaping, and limitations of saturable absorbers, and are also influenced by seemingly totally unrelated issues such as the beam quality of the available pump source.
For such reasons, a very systematic process of laser development, based on a solid quantitative understanding of all the relevant physical details and on deep experience with typical limitations, is essential for efficient product development. A key point is to work out a detailed laser design and to check quantitatively a number of issues before engaging in experimental investigations. Without such preparations, there is a risk of getting into a combination of problems which can not simply be solved step by step.
Some Special Achievements
Some special achievements with passively mode-locked solid-state lasers are:
- The very shortest pulses with durations below 10 fs (few-cycle pulses) are usually achieved with Kerr lens mode locking of a Ti:sapphire laser [6, 5].
- High average output powers of well over 200 W in sub-picosecond pulses [24, 25] and pulse energies above 10 μJ have been obtained in pulses from passively mode-locked thin-disk lasers [20, 19, 21], even 80 μJ in picosecond pulses [24].
- Very high pulse repetition rates have been obtained with passively mode-locked miniature bulk lasers [10, 15, 22] and also with harmonically mode-locked fiber lasers. Even higher values of > 1 THz are possible with small laser diodes [4].
- Various kinds of lasers (normally with high pulse repetition rates) have reached quantum-limited timing jitter performance, thus outperforming many high-quality electronic oscillators.
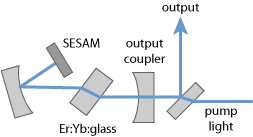
Higher Pulse Energies with Cavity Dumping
As explained in detail in the article on cavity dumping, a mode-locked laser can generate higher pulse energies of e.g. several microjoules at lower pulse repetition rates (e.g. 100 kHz or 1 MHz) by incorporation of a cavity dumper in the laser resonator. The basic principle is to form a high-energy pulse within the resonator while having low resonator losses, and then to couple out of the energy with the cavity dumper.
Typical Applications of Mode-locked Lasers
The following list gives some impression of the manifold applications of mode-locked lasers:
- Short pulses allow for time-resolved measurements, e.g. electro-optic sampling measurements on integrated electronic circuits, or pump–probe measurements on semiconductor devices such as SESAMs.
- Various methods of imaging, laser microscopy and laser spectroscopy greatly profit from short pulses for various reasons. For example, the high peak powers of femtosecond lasers are useful in two-photon absorption fluorescence microscopes, reaching a very high spatial resolution in all three dimensions.
- In the field of optical metrology, mode-locked lasers can be used for distance measurements, but also in frequency metrology (time keeping) and other fields. In the context of frequency metrology, the frequency combs of mode-locked lasers play a particularly important role.
- A number of processes for nonlinear frequency conversion are greatly facilitated by the high peak powers of mode-locked lasers, even when the average power remains moderate.
- Other fields with a large potential are microwave, millimeter-wave and terahertz optics, and picosecond optoelectronics.
Mode-locked lasers are also often combined with ultrafast amplifiers for obtaining higher average powers and in particular higher pulse energies and peak powers. Such amplified systems can address a wide range of additional applications:
- The high pulse intensities are used for applications in material processing, such as micromachining, surface treatment, drilling holes, and three-dimensional laser prototyping.
- In the medical domain, mode-locked lasers may again be used for a kind of material processing, e.g. as a laser scalpel or in ophthalmology (e.g. vision correction). There are also photochemical effects used e.g. for certain skin treatments.
- High-power laser projection displays may be realized with mode-locked lasers and frequency conversion stages, the latter often being much simpler when working with ultrashort pulses.
- High intensity physics relies on amplified systems with very high pulse energies and peak powers, so that extremely high optical intensities are achieved when focusing that laser radiation down to small spots.
Find more details in the articles on ultrafast lasers and ultrafast amplifiers.
Suppliers
The RP Photonics Buyer's Guide contains 48 suppliers for mode-locked lasers. Among them:
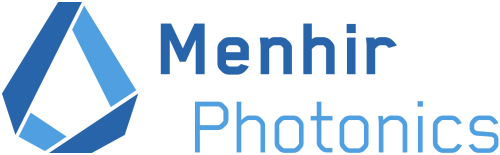
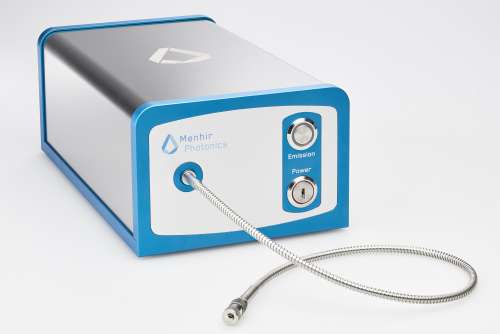
Menhir Photonics
Menhir Photonics offers ultrafast mode-locked lasers at 1.5 μm wavelength. These lasers offer pulse width below 200 fs and fundamental pulse repetition rates that can be chosen from 250 MHz up to 2.5 GHz. These systems are hermetically sealed and all-in-one (laser and electronic is one box). Menhir Photonics’ products have been designed to achieve ultra-low-noise performances combined with high-reliability and robustness, to ensure that they can be used in any situation from laboratory setup to harsh environments.
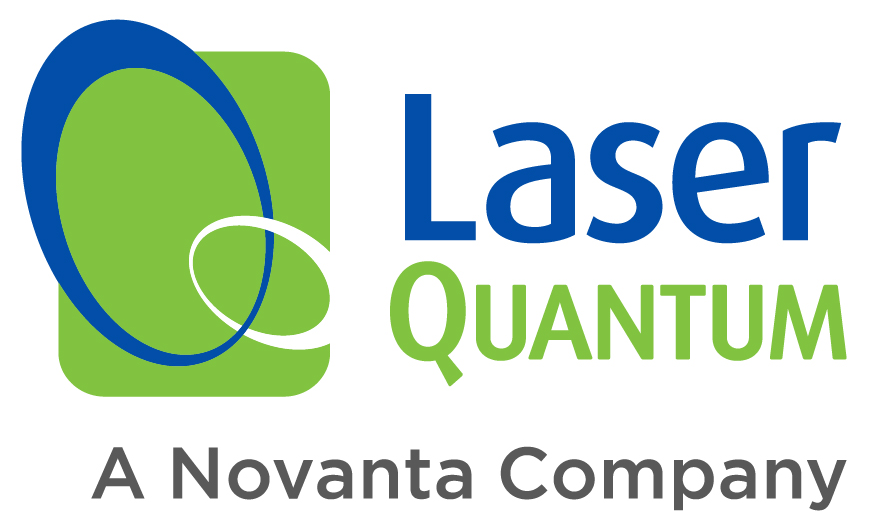
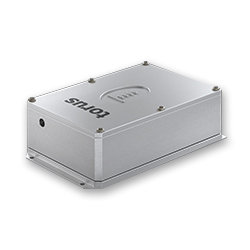
Laser Quantum
Laser Quantum are the manufacturers of the torus mode-locked SLM lasers, with patented active mode-locking technology to maintain the performance of the output beam, and a stable frequency output across wide temperature fluctuations and long measurement periods.
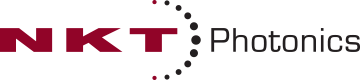
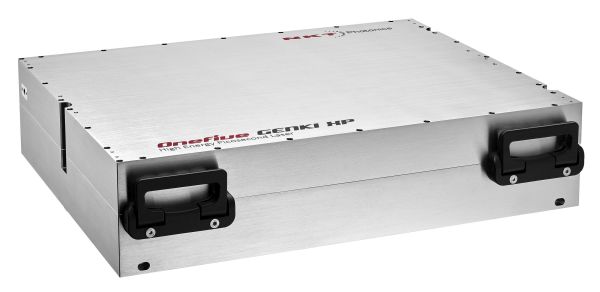
NKT Photonics
NKT Photonics passively mode-locked lasers, for industrial and scientific use, exhibit a compact packaging and the lowest phase noise on the market. A special hybrid laser setup, consisting of state-of-the-art polarization maintaining (PM) fiber technology and advanced dispersion control, allows for high pulse energy generation while supporting perfectly transform-limited soliton pulses without Kelly sidebands, temporal pedestals or satellite pulses. NKT Photonics lasers have been designed for high precision applications requiring long-term amplitude stability, low phase noise and timing jitter and 24/7 maintenance-free operation.
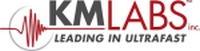
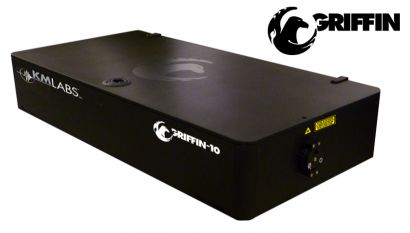
Kapteyn-Murnane Laboratories
The KMLabs Griffin™ series of Ti:sapphire oscillators gives customers ultimate control over their system, are simple to maintain, and offer a wide range of performance specifications enabling many different applications. These prism-based oscillators use Kerr lens modelocking to generate ultrashort <12 fs pulses. All Griffin lasers include computerized control of the spectral bandwidth and center wavelength and water-cooled breadboards for maximum long term stability. There are options within the Griffin series that include integrated pump lasers and diagnostics. Griffin lasers are very simple to maintain, since components are easily accessible.
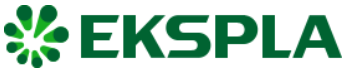
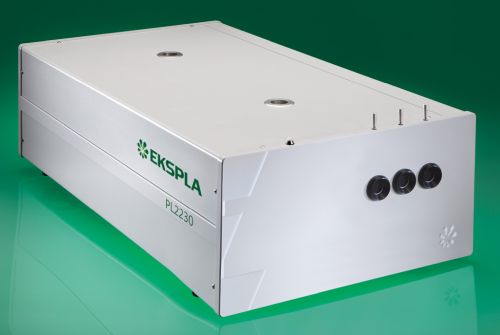
EKSPLA
Due to their excellent stability and high output parameters, EKSPLA scientific picosecond lasers established their name as “Gold Standard” among scientific picosecond lasers. The innovative design of the new generation of picosecond mode-locked lasers features diode-pumping‑only technology, thus reducing maintenance costs and improving output parameters. Second, third, fourth and fifth (on some versions) harmonic options combined with various accessories, advanced electronics (for streak camera synchronization, phase-locked loop, synchronization of fs laser) and customization possibilities make these lasers well suited for many scientific applications, including optical parametric generator pumping, time-resolved spectroscopy, nonlinear spectroscopy, remote sensing, metrology and others.
Questions and Comments from Users
Here you can submit questions and comments. As far as they get accepted by the author, they will appear above this paragraph together with the author’s answer. The author will decide on acceptance based on certain criteria. Essentially, the issue must be of sufficiently broad interest.
Please do not enter personal data here; we would otherwise delete it soon. (See also our privacy declaration.) If you wish to receive personal feedback or consultancy from the author, please contact him e.g. via e-mail.
By submitting the information, you give your consent to the potential publication of your inputs on our website according to our rules. (If you later retract your consent, we will delete those inputs.) As your inputs are first reviewed by the author, they may be published with some delay.
Bibliography
[1] | F. Krausz et al., “Femtosecond solid-state lasers”, IEEE J. Quantum Electron. 28 (10), 2097 (1992), doi:10.1109/3.159520 |
[2] | C. Spielmann et al., “Ultrabroadband femtosecond lasers”, IEEE J. Quantum Electron. 30 (4), 1100 (1994), doi:10.1109/3.291379 |
[3] | U. Keller, “Ultrafast all-solid-state laser technology”, Appl. Phys. B 58, 347 (1994), doi:10.1007/BF01081874 |
[4] | S. Arahira et al., “Mode-locking at very high repetition rates more than terahertz in passively mode-locked distributed-Bragg-reflector laser diodes”, IEEE J. Quantum Electron. 32 (7), 1211 (1996), doi:10.1109/3.517021 |
[5] | U. Morgner et al., “Sub-two cycle pulses from a Kerr-lens mode-locked Ti:sapphire laser”, Opt. Lett. 24 (6), 411 (1999), doi:10.1364/OL.24.000411 |
[6] | D. H. Sutter et al., “Semiconductor saturable-absorber mirror-assisted Kerr lens modelocked Ti:sapphire laser producing pulses in the two-cycle regime”, Opt. Lett. 24 (9), 631 (1999), doi:10.1364/OL.24.000631 |
[7] | C. Hönninger et al., “Ultrafast ytterbium-doped bulk lasers and laser amplifiers”, Appl. Phys. B 69 (1), 3 (1999), doi:10.1007/s003400050762 |
[8] | R. Paschotta et al., “Progress on all-solid-state passively mode-locked ps and fs lasers”, Proc. SPIE 3616, 2 (1999), doi:10.1117/12.351820 |
[9] | E. Sorokin et al., “Diode-pumped ultra-short-pulse solid-state lasers”, Appl. Phys. B 72, 3 (2001), doi:10.1007/s003400000464 |
[10] | L. Krainer et al., “Compact Nd:YVO4 lasers with pulse repetition rates up to 160 GHz”, IEEE J. Quantum Electron. 38 (10), 1331 (2002), doi:10.1109/JQE.2002.802967 |
[11] | U. Keller, “Recent developments in compact ultrafast lasers”, Nature 424, 831 (2003), doi:10.1038/nature01938 |
[12] | E. Innerhofer et al., “60 W average power in 810-fs pulses from a thin-disk Yb:YAG laser”, Opt. Lett. 28 (5), 367 (2003), doi:10.1364/OL.28.000367 |
[13] | A. Fernandez et al., “Chirped-pulse oscillators: a route to high-power femtosecond pulses without external amplification”, Opt. Lett. 29 (12), 1366 (2004), doi:10.1364/OL.29.001366 |
[14] | F. Brunner et al., “Powerful RGB laser source pumped with a mode-locked thin-disk laser”, Opt. Lett. 29 (16), 1921 (2004), doi:10.1364/OL.29.001921 |
[15] | S. C. Zeller et al., “Passively mode-locked 50-GHz Er:Yb:glass laser”, Electron. Lett. 40 (14), 875 (2004), doi:10.1049/el:20045064 |
[16] | R. Paschotta et al., “Picosecond pulse sources with multi-GHz repetition rates and high output power”, New J. Phys. 6, 174 (2004) |
[17] | E. Sorokin and S. Naumov, “Ultrabroadband infrared solid-state lasers”, J. Sel. Top. Quantum Electron. 11 (3), 690 (2005), doi:10.1109/JSTQE.2003.850255 |
[18] | S. Gee et al., “Self-stabilization of an actively mode-locked semiconductor-based fiber-ring laser for ultralow jitter”, IEEE Photon. Technol. Lett. 19 (7), 498 (2007), doi:10.1109/LPT.2007.892902 |
[19] | S. V. Marchese et al., “Femtosecond thin disk laser oscillator with pulse energy beyond the 10-microjoule level”, Opt. Express 16 (9), 6397 (2008), doi:10.1364/OE.16.006397 |
[20] | J. Neuhaus et al., “Passively mode-locked Yb:YAG thin-disk laser with pulse energies exceeding 13 μJ by use of an active multipass geometry”, Opt. Lett. 33 (7), 726 (2008), doi:10.1364/OL.33.000726 |
[21] | J. Neuhaus et al., “Subpicosecond thin-disk laser oscillator with pulse energies of up to 25.9 microjoules by use of an active multipass geometry”, Opt. Express 16 (25), 20530 (2008), doi:10.1364/OE.16.020530 |
[22] | A. E. Oehler et al., “100 GHz passively mode-locked Er:Yb:glass laser at 1.5 μm with 1.6-ps pulses”, Opt. Express 16 (26), 21930 (2008), doi:10.1364/OE.16.021930 |
[23] | P. Sévillano et al., “32-fs Kerr-lens mode-locked Yb:CaGdAlO4 oscillator optically pumped by a bright fiber laser”, Opt. Lett. 39 (20), 6001 (2014), doi:10.1364/OL.39.006001 |
[24] | C. J. Saraceno et al., “Ultrafast thin-disk laser with 80 μJ pulse energy and 242 W of average power”, Opt. Lett. 39 (1), 9 (2014), doi:10.1364/OL.39.000009 |
[25] | J. Brons et al., “Energy scaling of Kerr-lens mode-locked thin-disk oscillators”, Opt. Lett. 39 (22), 6442 (2014), doi:10.1364/OL.39.006442 |
[26] | R. Paschotta and U. Keller, “Passively mode-locked solid-state lasers”, in Solid-State Lasers and Applications (ed. A. Sennaroglu), CRC Press, Boca Raton, FL (2007), Chapter 7, pp. 259–318 |
[27] | R. Paschotta, Field Guide to Laser Pulse Generation, SPIE Press, Bellingham, WA (2007) |
[28] | For German readers: R. Paschotta, “Ultrakurzpuls-Festkörperlaser – eine vielfältige Familie”, Photonik 01/2006, S. 70 |
See also: ultrafast lasers, mode locking, mode locking devices, picosecond lasers, femtosecond lasers, titanium–sapphire lasers, mode-locked fiber lasers, mode-locked diode lasers, saturable absorbers, frequency combs, frequency metrology, pulses, ultrashort pulses, cavity dumping, timing jitter, laser design, laser development, The Photonics Spotlight 2008-03-26, The Photonics Spotlight 2010-03-22, The Photonics Spotlight 2010-07-27
and other articles in the categories lasers, light pulses

This encyclopedia is authored by Dr. Rüdiger Paschotta, the founder and executive of RP Photonics Consulting GmbH. How about a tailored training course from this distinguished expert at your location? Contact RP Photonics to find out how his technical consulting services (e.g. product designs, problem solving, independent evaluations, training) and software could become very valuable for your business!
![]() |
If you like this page, please share the link with your friends and colleagues, e.g. via social media:
These sharing buttons are implemented in a privacy-friendly way!