Fiber Amplifiers
This is part 6 of a tutorial on fiber amplifiers from Dr. Paschotta. The tutorial has the following parts:
1: Rare earth ions in fibers, 2: Gain and pump absorption, 3: Self-consistent solutions for the steady state, 4: Amplified spontaneous emission, 5: Forward and backward pumping, 6: Double-clad fibers for high-power operation, 7: Fiber amplifiers for nanosecond pulses, 8: Fiber amplifiers for ultrashort pulses, 9: Noise of fiber amplifiers, 10: Multi-stage fiber amplifiers
Part 6: High-power Fiber Amplifiers with Double-clad Fibers
Fiber amplifiers based on single-mode fibers are quite limited in output power, since it is hard to inject a high pump power into such a fiber. This has two reasons:
- The beam quality of high-power laser diodes is usually quite poor – insufficient for efficient launching into a single-mode core.
- Even if one had a high-power single-mode pump source, it would be a quite delicate issue to get that pump light injected. The high pump intensity in the fiber core would not be the main problem: after all, high-power fiber amplifiers can also cope with similarly high signal output powers. However, as the launch efficiency is never 100%, even with a high-quality pump beam, substantial power would be launched into the cladding and could then destroy the fiber by overheating, e.g. due to absorption at the coating (even if the fiber ends are stripped over some significant length).
That problem can be solved by using double-clad fibers. These have a pump cladding around the fiber core, which is itself surrounded by an outer cladding of even lower refractive index. The pump cladding, often having a substantially larger diameter than the fiber core and also a higher numerical aperture, constitutes a multimode waveguide into which high-power pump light can be launched easily and efficiently, even if the pump beam quality is not that great. The refractive index of the core is still higher than that of the pump cladding, so that it supports a single guided mode, or sometimes a few modes.
Light launched into the pump cladding also gets into the fiber core, where it can be absorbed by laser-active ions. (Note that the pump cladding is undoped, so there is no pump absorption there.) Only, the overlap of pump light with the doped core is reduced, as much of the pump power travels in the undoped pump cladding.
Figure 1 shows how pump light is injected into the inner cladding (pump cladding), while signal light is injected into the fiber core and remains there.
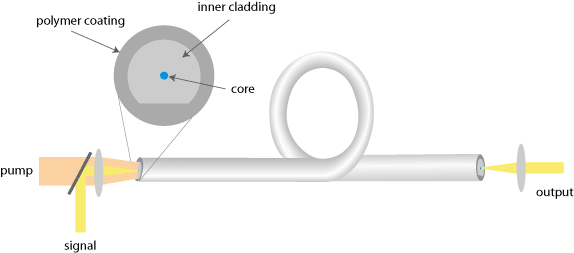
Optimizing the Pump Absorption
The mentioned reduced absorption of pump light injected into the pump cladding (instead of directly into the core) can lead to disadvantages, as are discussed in more detail below. Therefore, it is often beneficial to maximize the pump absorption in a double-clad fiber.
A straight-forward method is to make the pump cladding cladding as small as possible. This, of course, increases the constraints on the pump beam quality. The higher the numerical aperture (i.e., the index contrast between pump cladding and outer cladding), the less severe is that problem.
Another method is to make the doped fiber core as large as possible, so that the area ratio between pump cladding and core is reduced. The limits are often set by the need to have robust single-mode signal propagation, which is more difficult for large cores (see the encyclopedia article on large mode area fibers). Sometimes, one accepts having a several guided modes, although that makes it more difficult to obtain a high beam quality of the signal output, and can also lead to certain mode instabilities at high power levels.
Next, one can maximize the doping concentration of the fiber core. However, there are limits to that; at high doping concentrations, detrimental quenching effects become too strong, and for high-power operation one might get a too higher power dissipation per meter of fiber.
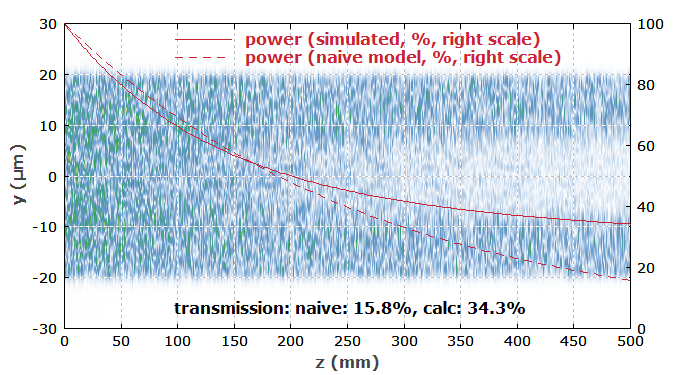
A nasty problem with some double-clad fibers is that the pump absorption is even substantially worse than one would expect simply by considering the above mentioned area ratio. This is because the overlap with the core is quite different for different modes of the pump cladding. Some of these have very little overlap with the core and thus exhibit only very weak pump absorption. After some length of fiber, substantial pump power may be left only in these modes, and it doesn't help much to make the fiber longer (see Figure 2). It also doesn't help that some of the pump cladding modes are absorbed much better than the average, since more than 100% absorption is anyway not possible.
There are various possible countermeasures, such as strong coiling of the fiber (which leads to mode scrambling) and using a less symmetric fiber design, which has no pump cladding modes with very low core overlap. Figure 3 shows different designs. The simplest kind of design with a circular pump cladding and a centered core, as simulated in Figure 2, is quite poor in terms of pump absorption, and the others are all better.

Double-clad fibers can also be made as photonic crystal fibers as shown in Figure 4. Here, the multimode pump core is suspended by very thin struts in the air cladding, through which the pump light cannot escape. Such a structure can have a very high numerical aperture for the pump light, which reduces the requirements concerning the pump beam quality. The guidance of the core is achieved as in other photonic crystal fibers.
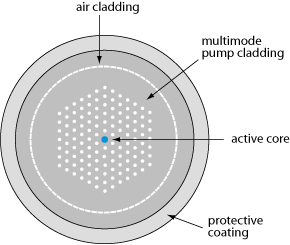
Limitations of Cladding Pumping
It would be quite wrong to expect that double-clad fibers allow essentially the same performance of fiber amplifiers, only at a higher power level. There are various issues, which we discuss in the following.
A first problem is a direct consequence of the reduced pump absorption: we need a substantially longer length of fiber, which can have various detrimental effects:
- The influence of parasitic propagation losses becomes stronger. That, however, is usually not a big problem. Typical losses of the order of 0.01 dB/m, for example, for ytterbium-doped double-clad fibers, amount only to 0.2 dB in 20 m, for example. That corresponds to a moderate power loss of 4.5%.
- Nonlinear effects become stronger. This is often an issue in the context of pulse amplification (see part 7).
- Compared with a core-pumped fiber, one has overall substantial more of the laser-active dopant in the core. As the interaction of the signal wave with the dopant is not reduced, this can have detrimental consequences (see the example case discussed below).
- Another issue can be that the pump intensity is relatively low in a double-clad fiber. That can be a problem if a high excitation density is required, e.g. in order to realize operation at relatively short wavelengths.
On the other hand, a certain fiber length is required to avoid overheating. At least, a much reduced fiber length would require aggressive cooling during high-power operation. Still, it would often be useful – particularly for pulse amplification – if the pump absorption could be improved further. That is particularly the case for erbium-doped fibers, as erbium ions have lower absorption cross-sections and a higher tendency for clustering effects, setting lower limits for the doping concentration.
Example: Ytterbium-doped Double-clad Fiber Amplifier
As an example, we consider an ytterbium-doped fiber which has the same core as in the previous examples, but pumping is done into a pump cladding around it with a 10 times larger diameter. The pump power at 940 nm is increased from 500 mW to 20 W, and the signal input power at 1030 nm from 1 mW to 40 mW. So both are 40 times higher than before, while the pump area is 100 times larger. The fiber is made 50 m long in order to provide good enough pump absorption. For simplicity, we assume perfect mode mixing in the fiber, so that the above explained problem of incomplete pump absorption does not occur; this is somewhat optimistic.
One might then hope to get the same nice performance as before, just with 40 times higher powers. That is not the case, however, as can be seen in Figure 5: after ≈60% of the pump power have been converted to signal power, the signal power begins to drop. So a longer fiber length would not help, even though the pump absorption is not yet perfectly efficient.
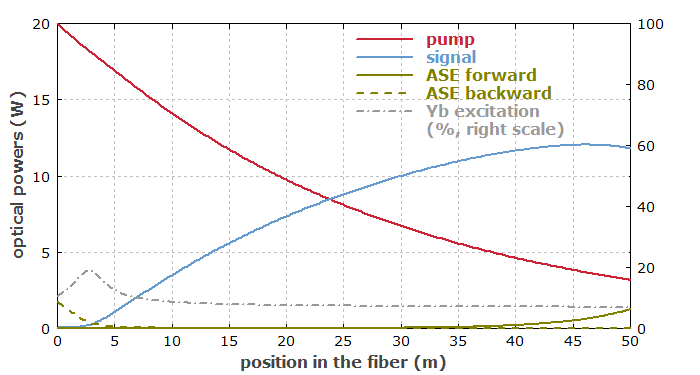
The main reason for that is that ASE comes up at somewhat longer wavelengths:
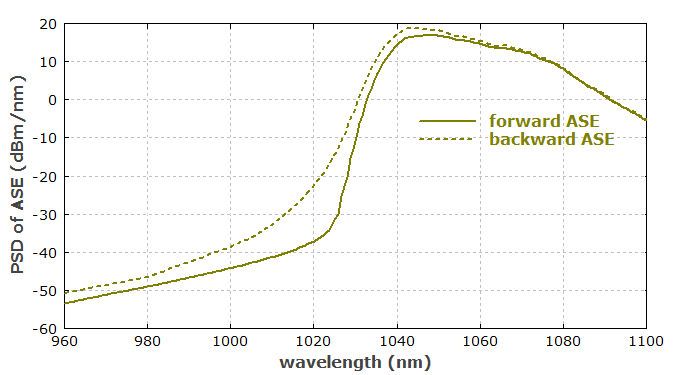
ASE can be largely suppressed by providing 10 times more signal input power, i.e., by operating the amplifier with a lower gain. Even then, however, the power conversion efficiency is limited to 73%. And if we use a slightly overlong fiber with 60 m, we again get a higher ASE-related power loss, and the conversion efficiency drops to 54%.
The amplifier would work better with a longer signal wavelength of 1080 nm, for example, as the signal wavelength is then closer to the wavelength of maximum gain. Indeed, one can find that high-power double-clad amplifiers and lasers are often operated at substantially longer wavelengths than core-pumped devices, even though this of course increases the quantum defect, which is particularly bad for high-power operation.
Example: Ytterbium-doped Double-clad Fiber Amplifier for 975 nm
As another example, we try to modify our amplifier such that it can amplify signals at 975 nm. As this is the wavelength where we got a lot of ASE in an example case of part 4, one could expect to easily get a lot of gain there when pumping at 940 nm. However, that doesn't work at all, as one can see in Figure 7:
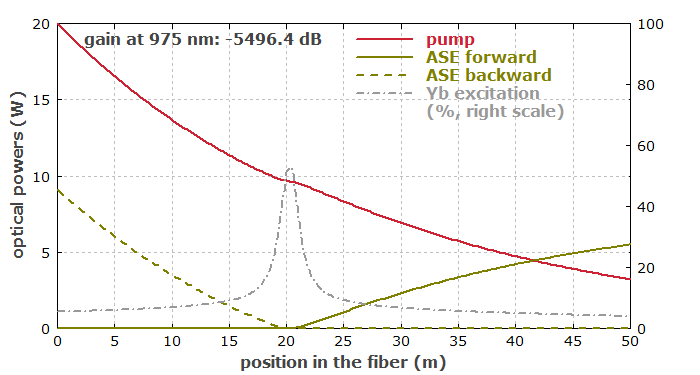
We produce a lot of forward and backward ASE, which peaks at 1075 nm in forward direction and at 1035 nm in backward direction (see Figure 8). At 975 nm, there is a huge absorption of nearly 5500 dB. (The absorption coefficient at 975 nm is rather high, and we have a lot of ytterbium in the fiber.) Stronger pumping would not help; we would only produce more long-wavelength ASE.
The problem is that if the average Yb excitation rises, we get a lot of gain in the long-wavelength region (generating strong ASE) long before we have 50% excitation, where the positive gain at 975 nm starts. That huge long-wavelength gain essentially results from the fact that we have a large amount of Yb in our fiber. In principle, a much shorter fiber would help: with 3 m instead of 50 m, we could get 33 dB gain at 975 nm for 20 W of pump power. However, the pump absorption is then very inefficient, so we could convert only a small part of the pump power into signal power at 975 nm.
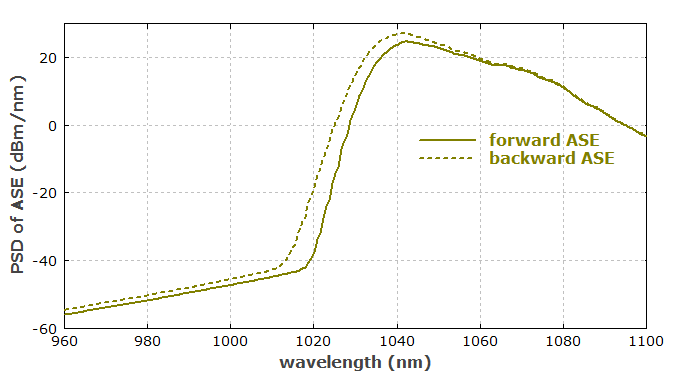
The problem can be summarized as follows:
- Good pump absorption despite the limited pump overlap with the core requires that we put a lot of laser-active dopant into the fiber core.
- With so much dopant, a small average excitation density is sufficient to create a large long-wavelength gain, while it is by far not sufficient to provide gain at shorter wavelengths.
There is actually a solution to that: using a ring-doped fiber (J. Nilsson et al., Opt. Lett. 22 (14), 1092 (1997)). That reduces the coupling of the dopant to the ASE and signal waves, but not to the pump light. In effect, one can have higher excitation densities, allowing for gain at shorter wavelengths.
Some Conclusions
We have seen that it is not always easy to get the same kind of performance of an amplifier at much higher power levels simply be using double-clad fibers. One can run into trouble with unwanted ASE and/or incomplete pump absorption, for example. The findings are often surprising. Clearly, a trial-and-error approach in the lab, which is not based on a thorough understanding as obtained with numerical simulation, would be fishing in the dark: test devices would not work, and it would not be obvious in the lab why not.
So far, we consider only continuous-wave operation. Next we will look at the amplification of pulses.
Go to Part 7: Fiber Amplifiers for Nanosecond Pulses or back to the start page.
Questions and Comments from Users
Here you can submit questions and comments. As far as they get accepted by the author, they will appear above this paragraph together with the author’s answer. The author will decide on acceptance based on certain criteria. Essentially, the issue must be of sufficiently broad interest.
Please do not enter personal data here; we would otherwise delete it soon. (See also our privacy declaration.) If you wish to receive personal feedback or consultancy from the author, please contact him e.g. via e-mail.
By submitting the information, you give your consent to the potential publication of your inputs on our website according to our rules. (If you later retract your consent, we will delete those inputs.) As your inputs are first reviewed by the author, they may be published with some delay.



These sharing buttons are implemented in a privacy-friendly way!